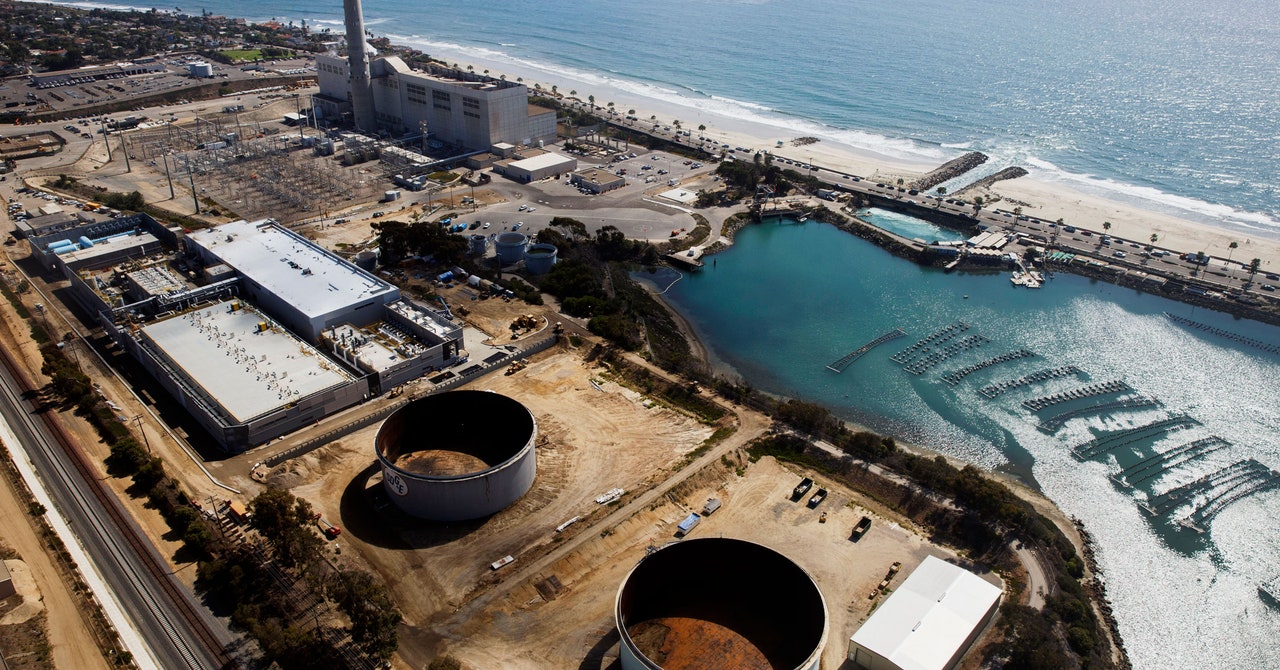
Friction is resistance. In this case, it tells you how hard it is for something to get across the membrane. If you engineer a membrane that has less resistance to water, and more resistance to salt or whatever else you want to remove, you get a cleaner product with potentially less work.
But that model got shelved in 1965, when another group introduced a simpler model. This one assumed that the plastic polymer of the membrane was dense and had no pores through which water could run. It also didn’t hold that friction played a role. Instead, it presumed that water molecules in a saltwater solution would dissolve into the plastic and diffuse out of the other side. For that reason, this is called the “solution-diffusion” model.
Diffusion is the flow of a chemical from where it’s more concentrated to where it’s less concentrated. Think of a drop of dye spreading throughout a glass of water, or the smell of garlic wafting out of a kitchen. It keeps moving toward equilibrium until its concentration is the same everywhere, and it doesn’t rely on a pressure difference, like the suction that pulls water through a straw.
The model stuck, but Elimelech always suspected it was wrong. To him, accepting that water diffuses through the membrane implied something strange: that the water scattered into individual molecules as it passed through. “How can it be?” Elimelech asks. Breaking up clusters of water molecules requires a ton of energy. “You almost need to evaporate the water to get it into the membrane.”
Still, Hoek says, “20 years ago it was anathema to suggest that it was incorrect.” Hoek didn’t even dare to use the word “pores” when talking about reverse osmosis membranes, since the dominant model didn’t acknowledge them. “For many, many years,” he says wryly, “I’ve been calling them ‘interconnected free volume elements.’”
Over the past 20 years, images taken using advanced microscopes have reinforced Hoek and Elimelech’s doubts. Researchers discovered that the plastic polymers used in desalination membranes aren’t so dense and poreless after all. They actually contain interconnected tunnels—although they are absolutely minuscule, peaking at around 5 angstroms in diameter, or half a nanometer. Still, one water molecule is about 1.5 angstroms long, so that’s enough room for small clusters of water molecules to squeeze through these cavities, instead of having to go one at a time.
About two years ago, Elimelech felt the time was right to take down the solution-diffusion model. He worked with a team: Li Wang, a postdoc in Elimelech’s lab, examined fluid flow through small membranes to take real measurements. Jinlong He, at the University of Wisconsin-Madison, tinkered with a computer model simulating what happens at the molecular scale as pressure pushes salt water through a membrane.
Predictions based on a solution-diffusion model would say that water pressure should be the same on both sides of the membrane. But in this experiment, the team found that the pressure at the entrance and exit of the membrane differed. This suggested that pressure drives water flow through the membrane, rather than simple diffusion.