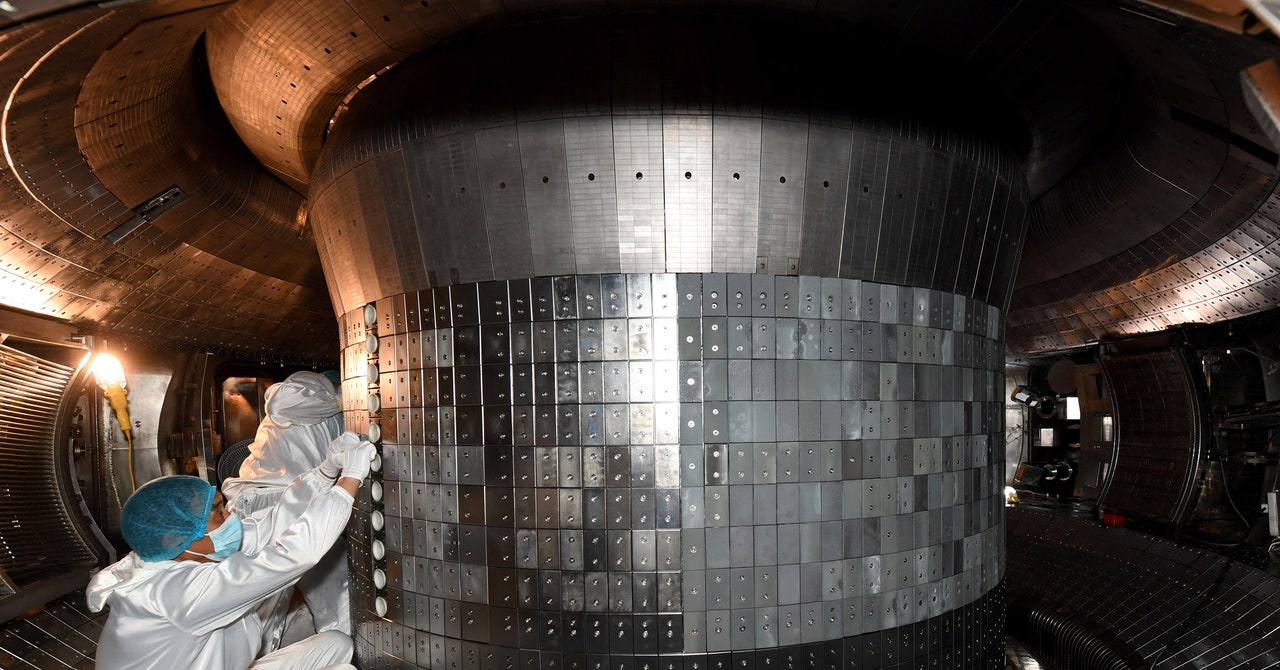
There are a few hundred such reactors, known as tokamaks, in state-funded research facilities around the world, including the Joint European Torus in the United Kingdom, and ITER, the International Thermonuclear Experimental Reactor, a 35-nation collaboration in southern France. For decades, researchers have been using them to grapple with the challenges of nuclear fusion, a potentially revolutionary technology that could provide essentially unlimited power. Inside a tokamak, powerful magnets are used to hold whirling plasma at a high pressure, enabling it to reach the tens of millions of degrees required for atoms to fuse together and release energy. Cynics argue that nuclear fusion is doomed to forever remain the energy source of the future—right now, fusion experiments still consume more electricity than they generate.
But Kostadinova and her collaborator Dimitri Orlov were more interested in the plasma inside these reactors, which they realized could be the perfect environment to simulate a spacecraft entering the atmosphere of a gas giant. Orlov works on the DIII-D fusion reactor, an experimental tokamak at a US Department of Energy facility in San Diego, but his background is in aerospace engineering.
Together, they used the DIII-D facilities to run a series of experiments on ablation. Using a port at the bottom of the tokamak, they inserted a series of carbon rods into the plasma flow, and used high-speed and infrared cameras and spectrometers to track how they disintegrated. Orlov and Kostadinova also fired minuscule carbon pellets into the reactor at high speed, mimicking on a small scale what the heat shield on the Galileo probe would have encountered in Jupiter’s atmosphere.
The conditions inside the tokamak were remarkably similar in terms of the temperature of the plasma, the speed it flowed over the material, and even its composition: The Jovian atmosphere is mostly hydrogen and helium, the DIII-D tokamak uses deuterium, which is an isotope of hydrogen. “Instead of launching something at a very high velocity, we instead put a stationary object into a very fast flow,” Orlov says.
The experiments, which were presented at a meeting of the American Physical Society in Pittsburgh this month, helped to validate the models of ablation that were developed by NASA scientists using data sent back from the Galileo probe. But they also serve as a proof of concept for a new type of testing. “We’re opening this new field of research,” says Orlov. “Nobody has done it before.”
It’s something that’s sorely needed in the industry. “There’s been a lag in new testing procedures,” says Yanni Barghouty, founder of Cosmic Shielding Corporation, a startup building radiation shields for spacecraft. “It allows you to prototype a lot faster and more cheaply—there’s a feedback loop.”
Whether nuclear fusion reactors will be a practical testing ground remains to be seen—they’re incredibly sensitive devices that have been designed for another purpose entirely. Orlov and Kostadinov were given time at DIII-D as part of a special effort to use the reactor to expand scientific knowledge, utilizing a port built into the tokamak for the purpose of safely testing new materials. But it’s an expensive process. Their day on the machine cost half a million dollars. As a result, this kind of experiment will likely be done sparingly in the future, when the opportunity arises, to tweak and improve computer simulations.