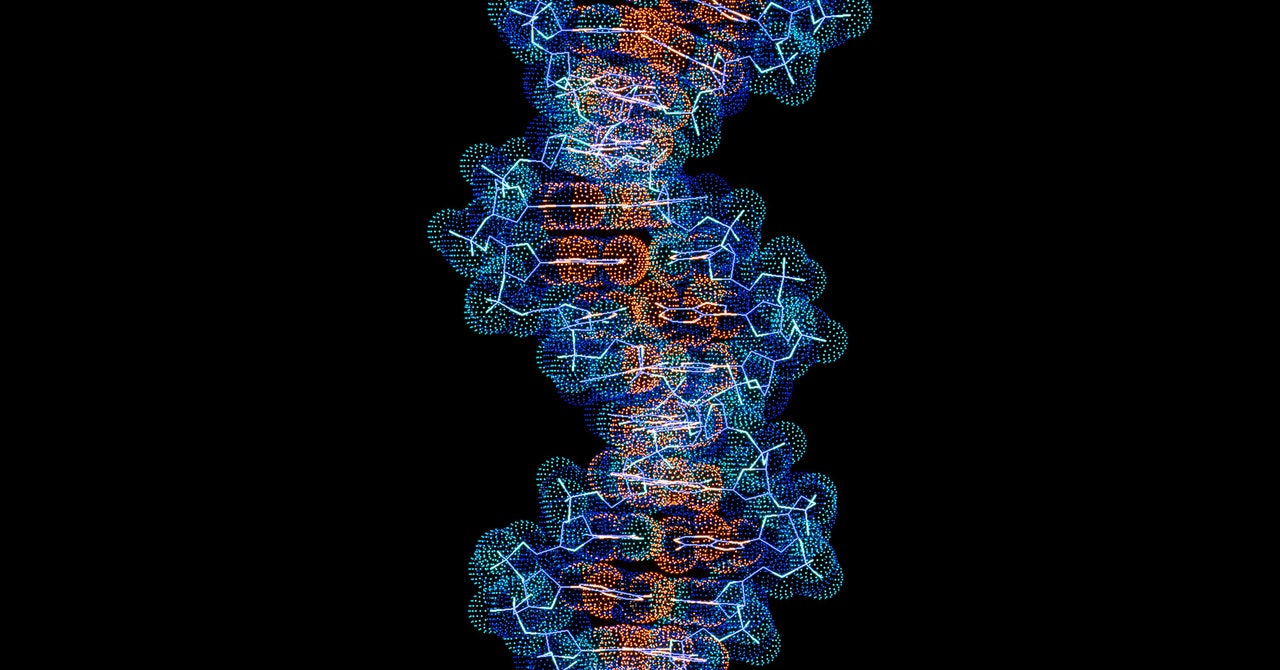
Faced with a threat, the brain has to act fast, its neurons making new connections to learn what might spell the difference between life and death. But in its response, the brain also raises the stakes: As an unsettling recent discovery shows, to express learning and memory genes more quickly, brain cells snap their DNA into pieces at many key points, and then rebuild their fractured genome later.
The finding doesn’t just provide insights into the nature of the brain’s plasticity. It also demonstrates that DNA breakage may be a routine and important part of normal cellular processes—which has implications for how scientists think about aging and disease, and how they approach genomic events they’ve typically written off as merely bad luck.
The discovery is all the more surprising because DNA double-strand breaks, in which both rails of the helical ladder get cut at the same position along the genome, are a particularly dangerous kind of genetic damage associated with cancer, neurodegeneration and aging. It’s more difficult for cells to repair double-strand breaks than other kinds of DNA damage because there isn’t an intact “template” left to guide the reattachment of the strands.
Yet it’s also long been recognized that DNA breakage sometimes plays a constructive role, too. When cells are dividing, double-strand breaks allow for the normal process of genetic recombination between chromosomes. In the developing immune system, they enable pieces of DNA to recombine and generate a diverse repertoire of antibodies. Double-strand breaks have also been implicated in neuronal development and in helping turn certain genes on. Still, those functions have seemed like exceptions to the rule that double-strand breaks are accidental and unwelcome.
But a turning point came in 2015. Li-Huei Tsai, a neuroscientist and director of the Picower Institute for Learning and Memory at the Massachusetts Institute of Technology, and her colleagues were following up on previous work that had linked Alzheimer’s disease with the accumulation of double-strand breaks in neurons. To their surprise, the researchers found that stimulating cultured neurons triggered double-strand breaks in their DNA, and the breaks quickly increased the expression of a dozen fast-acting genes associated with synaptic activity in learning and memory.
The double-strand breaks seemed to be essential for regulating gene activity important to the neurons’ function. Tsai and her collaborators hypothesized that the breaks essentially released enzymes that were stuck along twisted pieces of DNA, freeing them to transcribe relevant nearby genes quickly. But the idea “was met with a lot of skepticism,” Tsai said. “People simply have a hard time imagining that double-strand breaks can actually be physiologically important.”
Nevertheless, Paul Marshall, a postdoctoral researcher at the University of Queensland in Australia, and his colleagues decided to follow up on the finding. Their work, which appeared in 2019, both confirmed and extended the observations by Tsai’s team. It showed that the DNA breakage touched off two waves of enhanced gene transcription, one immediate and one several hours later.
Marshall and his colleagues proposed a two-step mechanism to explain the phenomenon: When the DNA breaks, some enzyme molecules are freed for transcription (as Tsai’s group suggested) and the site of the break is also chemically flagged with a methyl group, a so-called epigenetic marker. Later, when repair of the broken DNA begins, the marker is removed—and in the process, still more enzymes can spill free, starting the second round of transcription.
“Not only is the double-strand break involved as a trigger,” Marshall said, “it then becomes a marker, and that marker itself is functional in terms of regulating and guiding machinery to that location.”
Since then, other studies have demonstrated something similar. One, published last year, associated double-strand breaks not just with the formation of a fear memory, but with its recollection.
Now, in a study last month in PLOS ONE, Tsai and her colleagues have shown that this counterintuitive mechanism of gene expression might be prevalent in the brain. This time, instead of using cultured neurons, they looked at cells in the brains of living mice that were learning to associate an environment with an electric shock. When the team mapped genes undergoing double-strand breaks in the prefrontal cortex and hippocampus of mice that had been shocked, they found breaks occurring near hundreds of genes, many of which were involved in synaptic processes related to memory.